All MCAT Biology Resources
Example Questions
Example Question #21 : Biochemistry And Metabolism
The drug, DNP, destroys the H+ gradient that forms in the electron transport chain. What is the most likely consequence?
ATP production will increase.
Glycolysis will stop.
No effect will occur.
The cells will be forced to perform fermentation.
Oxygen consumption will increase.
The cells will be forced to perform fermentation.
If the proton gradient of the electron transport chain were to be destroyed, the cell would need to perform cellular respiration without an electron transport chain. The only option would be to move to anaerobic respiration, which requires fermentation.
Example Question #1701 : Mcat Biological Sciences
Given a healthy individual with a normal metabolic rate, which of the following compounds is the most energy rich?
GTP
FADH2
NADH
ATP
NADH
This question is asking about ATP production during cellular respiration. During oxidative phosphorylation (the electron transport chain), each 1 ATP is produced for each GTP, 2 ATP are produced for each FADH2, and 3 ATP are produced for each NADH.
Example Question #21 : Biochemistry And Metabolism
A person is born with a mutation that causes their cells to not have the ability to produce the NADH dehydrogenase complex, the complex that allows the electron transport chain to make ATP from NADH. Will this patient be able to produce any enery at all from the ETC?
No—FAD can still enter the ETC.
No—NADH is necessary for the ETC to use other molecules to make ATP.
No—there are no other molecules the ETC uses.
Yes—NAD+ can still enter the ETC.
Yes—FADH2 can still enter the ETC.
Yes—FADH2 can still enter the ETC.
FADH2 enters the ETC at the succinate-Q oxidoreductase complex. While this doesn't generate as much energy as NADH will because the electrons travel a shorter distance, there are still 2 ATP molecules made for each FADH2.
Example Question #22 : Biochemistry And Metabolism
Scientists use a process called Flourescent In-Situ Hybridization, or FISH, to study genetic disorders in humans. FISH is a technique that uses spectrographic analysis to determine the presence or absence, as well as the relative abundance, of genetic material in human cells.
To use FISH, scientists apply fluorescently-labeled bits of DNA of a known color, called probes, to samples of test DNA. These probes anneal to the sample DNA, and scientists can read the colors that result using laboratory equipment. One common use of FISH is to determine the presence of extra DNA in conditions of aneuploidy, a state in which a human cell has an abnormal number of chromosomes. Chromosomes are collections of DNA, the totality of which makes up a cell’s genome. Another typical use is in the study of cancer cells, where scientists use FISH labels to ascertain if genes have moved inappropriately in a cell’s genome.
Using red fluorescent tags, scientists label probe DNA for a gene known to be expressed more heavily in cancer cells than normal cells. They then label a probe for an immediately adjacent DNA sequence with a green fluorescent tag. Both probes are then added to three dishes, shown below. In dish 1 human bladder cells are incubated with the probes, in dish 2 human epithelial cells are incubated, and in dish 3 known non-cancerous cells are used. The relative luminescence observed in regions of interest in all dishes is shown below.
Cancer cells require large amounts of energy in the form of ATP. Which of the following processes results in the greatest production of ATP?
Oxidative phosphorylation
Substrate-level phosphorylation
The Krebs cycle
Fermentation
Glycolysis
Oxidative phosphorylation
Oxidative phosphorylation in the mitochondria is the major contributor to the total ATP pool in most eukaryotic cells. Keep in mind that it is oxidative phosphorylation in concert with the proton gradient that drives the electron transport chain.
Example Question #23 : Biochemistry And Metabolism
Scientists use a process called Flourescent In-Situ Hybridization, or FISH, to study genetic disorders in humans. FISH is a technique that uses spectrographic analysis to determine the presence or absence, as well as the relative abundance, of genetic material in human cells.
To use FISH, scientists apply fluorescently-labeled bits of DNA of a known color, called probes, to samples of test DNA. These probes anneal to the sample DNA, and scientists can read the colors that result using laboratory equipment. One common use of FISH is to determine the presence of extra DNA in conditions of aneuploidy, a state in which a human cell has an abnormal number of chromosomes. Chromosomes are collections of DNA, the totality of which makes up a cell’s genome. Another typical use is in the study of cancer cells, where scientists use FISH labels to ascertain if genes have moved inappropriately in a cell’s genome.
Using red fluorescent tags, scientists label probe DNA for a gene known to be expressed more heavily in cancer cells than normal cells. They then label a probe for an immediately adjacent DNA sequence with a green fluorescent tag. Both probes are then added to three dishes, shown below. In dish 1 human bladder cells are incubated with the probes, in dish 2 human epithelial cells are incubated, and in dish 3 known non-cancerous cells are used. The relative luminescence observed in regions of interest in all dishes is shown below.
A new treatment for bladder cancer is developed that targets energy production in malignant cells. Which of the following potential target sites would directly involve the synthesis of most of the ATP in a cell?
Ribosomes
ATP synthase, an enzyme housed on the outer mitochondrial membrane
ATP synthase, an enzyme housed on the inner mitochondrial membrane
Lactate dehydrogenase, an enzyme in the cytosol
ATP synthase, an enzyme housed in the mitochondrial matrix
ATP synthase, an enzyme housed on the inner mitochondrial membrane
ATP synthase is housed on the inner mitochondrial membrane, and is the main ATP production agent in oxidative phosphorylation. It provides a channel for protons to enter the matrix from the intermembrane space, and in so doing, drives ATP production.
Example Question #313 : Organic Chemistry, Biochemistry, And Metabolism
What phase of cellular respiration has the highest ATP yield?
Fermentation
Oxidative phosphorylation
Gluconeogenesis
Glycolysis
Krebs cycle
Oxidative phosphorylation
Oxidative phosphorylation, which traps energy in a high-energy phosphate bond and uses an electron gradient and ATP synthase to create ATP, yields the most ATP. Oxidative phosphorylation is linked with the electron transport chain.
Glycolysis only gives a net of two ATP per glucose, and the Krebs cycle gives two GTP for every turn of the cycle. Gluconeogenesis is not a part of cellular respiration, and fermentation is very low-yield since it occurs in the absence of oxygen.
Example Question #1 : Electron Transport Chain And Oxidative Phosphorylation
Why is oxygen necessary in aerobic cellular respiration?
It is important in creating oxaloacetic acid in the Kreb's cycle.
It is the final electron acceptor in the electron transport chain.
It is needed for glycolysis, which begins the process of respiration in cells.
It provides the hydrogen nuclei needed to create a proton gradient in the intermemberane space.
It is the final electron acceptor in the electron transport chain.
Oxygen is the final electron acceptor in the electron transport chain, which results in the production of water. Glycolysis does not require oxygen, and can be done in anaerobic environments. NADH is the molecule which is oxidized in order to establish the proton gradient. Finally, oxygen is not needed to create oxaloacetic acid is the Kreb's cycle, as it is regenerated after each turn of the cycle.
Example Question #25 : Biochemistry And Metabolism
Most scientists subscribe to the theory of endosymbiosis to explain the presence of mitochondria in eukaryotic cells. According to the theory of endosymbiosis, early pre-eukaryotic cells phagocytosed free living prokaryotes, but failed to digest them. As a result, these prokaryotes remained in residence in the pre-eukaryotes, and continued to generate energy. The host cells were able to use this energy to gain a selective advantage over their competitors, and eventually the energy-producing prokaryotes became mitochondria.
In many ways, mitochondria are different from other cellular organelles, and these differences puzzled scientists for many years. The theory of endosymbiosis concisely explains a number of these observations about mitochondria. Perhaps most of all, the theory explains why aerobic metabolism is entirely limited to this one organelle, while other kinds of metabolism are more distributed in the cellular cytosol.
One of the main arguments in favor of the theory of endosymbiosis is that mitochondria have their own genome. Which of the following cellular structures is most likely to be coded for only by mitochondrial DNA?
Glycolytic enzymes
Insulin-like growth factor 1
Sodium-potassium ATPase
Electron transport chain proteins
Growth hormone
Electron transport chain proteins
Electron transport chain (ETC) proteins are encoded by the the mitochondrial DNA. This makes sense, as we find ETC proteins only in the mitochondrial membrane (remember, as the passage states, aerobic metabolism is limited to the mitochondria).
It may be tempting to select glycolytic enzymes, as the free living predecessors to mitochondria presumably underwent glycolysis; however, these genes have been lost as the symbiosis matured and glycolysis was localized to the cellular cytosol.
Example Question #26 : Biochemistry And Metabolism
Most scientists subscribe to the theory of endosymbiosis to explain the presence of mitochondria in eukaryotic cells. According to the theory of endosymbiosis, early pre-eukaryotic cells phagocytosed free living prokaryotes, but failed to digest them. As a result, these prokaryotes remained in residence in the pre-eukaryotes, and continued to generate energy. The host cells were able to use this energy to gain a selective advantage over their competitors, and eventually the energy-producing prokaryotes became mitochondria.
In many ways, mitochondria are different from other cellular organelles, and these differences puzzled scientists for many years. The theory of endosymbiosis concisely explains a number of these observations about mitochondria. Perhaps most of all, the theory explains why aerobic metabolism is entirely limited to this one organelle, while other kinds of metabolism are more distributed in the cellular cytosol.
With regard to the energy production by the mitochondria discussed in the passage, what is the main factor driving ATP production at the terminal step of aerobic metabolism?
Energy from the electrochemical proton gradient is captured by ATP synthase
The oxidation of glucose to pyruvate
The reduction of glucose to pyruvate
The reduction of glucose to lactate
The oxidation of glucose to lactate
Energy from the electrochemical proton gradient is captured by ATP synthase
The final step in aerobic metabolism is the capture of the stored energy of protons existing in the intermembrane space. The electrochemical gradient in the intermembrane space forces protons through ATP synthase, phosphorylating ADP.
Glucose is converted to pyruvate during glycolysis, and to lactate during anaerobic respiration.
Example Question #27 : Biochemistry And Metabolism
Most scientists subscribe to the theory of endosymbiosis to explain the presence of mitochondria in eukaryotic cells. According to the theory of endosymbiosis, early pre-eukaryotic cells phagocytosed free living prokaryotes, but failed to digest them. As a result, these prokaryotes remained in residence in the pre-eukaryotes, and continued to generate energy. The host cells were able to use this energy to gain a selective advantage over their competitors, and eventually the energy-producing prokaryotes became mitochondria.
In many ways, mitochondria are different from other cellular organelles, and these differences puzzled scientists for many years. The theory of endosymbiosis concisely explains a number of these observations about mitochondria. Perhaps most of all, the theory explains why aerobic metabolism is entirely limited to this one organelle, while other kinds of metabolism are more distributed in the cellular cytosol.
The primary purpose of the electron transport chain of mitochondria described in the passage is __________.
to directly phosphorylate ADP
to carry ADP into the mitochondrial matrix
to directly phosphorylate AMP
to synthesize ATP synthase
the generation of energy to sequester protons in the intermembrane space
the generation of energy to sequester protons in the intermembrane space
The electron transport chain serves to pump protons into the intermembrane space. The result is the buildup of the electrochemical gradient, and the passage of protons through ATP synthase. Essentially, the electron transport chain establishes the conditions for oxidative phosphorylation to occur.
Certified Tutor
All MCAT Biology Resources
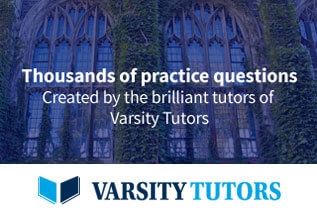